Linear geometry-frequency scalability in metamaterial absorbers
TÓM TẮT
TƯƠNG THÍCH HÌNH HỌC - TẦN SỐ TUYẾN TÍNH
CỦA VẬT LIỆU HẤP THỤ MEATMATERIALS
Tương thích tuyến tính của tần số với sự thay đổi của các thông số vật lý là một
ưu điểm quan trọng của vật liệu hấp thụ trong nhiều ứng dụng. Trong báo cáo này,
chúng tôi nghiên cứu khả năng hấp thụ đơn giản nhưng hiệu suất cao của siêu vật
liệu metamaterials, cho phép biến đổi tương cấu trúc - tần số một cách tuyến tính
trong dải tần số rộng. Mẫu vật liệu hấp thụ được chế tạo bằng phương pháp quang
khắc truyền thống, sau đó, đặc trưng hấp thụ được kiểm tra bằng phương pháp
phân tích mạng vector trong giải tần 8-12 GHz. Kỹ thuật mô phỏng tích phân hữu
hạn cũng được áp dụng để khẳng định các giá trị đo thực nghiệm.
Bạn đang xem tài liệu "Linear geometry-frequency scalability in metamaterial absorbers", để tải tài liệu gốc về máy hãy click vào nút Download ở trên
Tóm tắt nội dung tài liệu: Linear geometry-frequency scalability in metamaterial absorbers
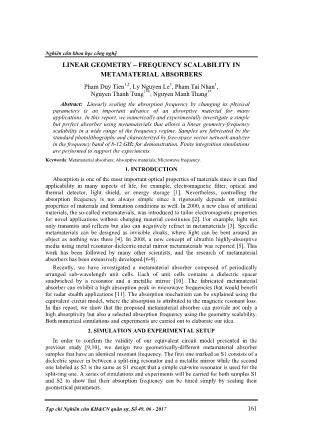
Nghiên cứu khoa học công nghệ Tạp chí Nghiên cứu KH&CN quân sự, Số 49, 06 - 2017 161 LINEAR GEOMETRY – FREQUENCY SCALABILITY IN METAMATERIAL ABSORBERS Pham Duy Tien1,2, Ly Nguyen Le3, Pham Tai Nhan1, Nguyen Thanh Tung1**, Nguyen Manh Thang3* Abstract: Linearly scaling the absorption frequency by changing its physical parameters is an important advance of an absorptive material for many applications. In this report, we numerically and experimentally investigate a simple but perfect absorber using metamaterials that allows a linear geometry-frequency scalability in a wide range of the frequency regime. Samples are fabricated by the standard photolithography and characterized by free-space vector network analyzer in the frequency band of 8-12 GHz for demonstration. Finite integration simulations are performed to support the experiments. Keywords: Metamaterial absorbers; Absorptive materials; Microwave frequency. 1. INTRODUCTION Absorption is one of the most important optical properties of materials since it can find applicability in many aspects of life, for example, electromagnetic filter, optical and thermal detector, light shield, or energy storage [1]. Nevertheless, controlling the absorption frequency is not always simple since it rigorously depends on intrinsic properties of materials and formation conditions as well. In 2000, a new class of artificial materials, the so-called metamaterials, was introduced to tailor electromagnetic properties for novel applications without changing material constitutes [2]. For example, light not only transmits and reflects but also can negatively refract in metamaterials [3]. Specific metamaterials can be designed as invisible cloaks, where light can be bent around an object as nothing was there [4]. In 2008, a new concept of ultrathin highly-absorptive media using metal resonator-dielectric-metal mirror metamaterials was reported [5]. This work has been followed by many other scientists, and the research of metamaterial absorbers has been extensively developed [6-9]. Recently, we have investigated a metamaterial absorber composed of periodically arranged sub-wavelength unit cells. Each of unit cells contains a dielectric spacer sandwiched by a resonator and a metallic mirror [10]. The fabricated metamaterial absorber can exhibit a high absorption peak in microwave frequencies that would benefit for radar stealth applications [11]. The absorption mechanism can be explained using the equivalent circuit model, where the absorption is attributed to the magnetic resonant loss. In this report, we show that the proposed metamaterial absorber can provide not only a high absorptivity but also a selected absorption frequency using the geometry scalability. Both numerical simulations and experiments are carried out to elaborate our idea. 2. SIMULATION AND EXPERIMENTAL SETUP In order to confirm the validity of our equivalent circuit model presented in the previous study [9,10], we design two geometrically-different metamaterial absorber samples that have an identical resonant frequency. The first one marked as S1 consists of a dielectric spacer in between a split-ring resonator and a metallic mirror while the second one labeled as S2 is the same as S1 except that a simple cut-wire resonator is used for the split-ring one. A series of simulations and experiments will be carried for both samples S1 and S2 to show that their absorption frequency can be tuned simply by scaling their geometrical parameters. Vật lý P.D. Tien, L.N. Le, , “Linear geometry-frequency scalability metamaterial absorbers.” 162 Figure 1. Computational unit cell of metamaterial absorbers and corresponding photos of their actual samples for (a) S1 and (b) S2. The metallic patterns and mirror are indicated by yellow while the dielectric material is blue. The geometrical parameters of fabricated samples are as follow: for S1, w= 1 mm, c = 3 mm, g = 2mm, b = 8 mm, and a = 12mm; for S2, w = 1 mm, l = 8.5 mm, and a = 12 mm. Figure 1 illustrates the schematic drawing of S1 and S2 with polarization and geometric parameters. The incident wave is normal to the structure plane while the electric and the magnetic directions are defined to be parallel to y-axis and x-axis respectively. In both experiments and simulations, the metal is chosen as copper while FR-4 is selected for dielectric material. The thicknesses of copper and dielectric components are tc=0.036 mm and td=0.4 mm, respectively. The electromagnetic simulations are performed by CST software using the finite-integration-simulation technique [12]. The unit cell is placed between two waveguide ports, which act like transmitter and also receiver antennas. For experiments, the metamaterial samples are fabricated using commercial FR4 printed circuit board (PCB) following unit-cell parameters aforementioned. Undergoing photo-lithography and chemical etching steps, the metamaterial perfect absorber is formed. During measurements, the samples are distanced between two horn antennas in a reflection mode and held by a homemade plastic sample holder. The reflection signal is recorded by a Vector Network Analyzer MS2028B. Since the transmission is prohibited by the metallic mirror, the absorption intensity is obtained via the corresponding reflection. 3. RESULTS AND DISCUSSIONS It has been agreed that the origin of metamaterial absorption associated with a magnetic resonance and its corresponding antiparallel current distribution [9]. The magnetic resonant frequency can be described by a LC equivalent circuit model f=1/[2 .sqrt(LC)], where C is the induced capacitance between two metallic layers, and L is the induced Nghiên cứu khoa học công nghệ Tạp chí Nghiên cứu KH&CN quân sự, Số 49, 06 - 2017 163 inductance of metallic resonators. In general, for each shape of the front resonator, i.e. split-ring [5], disk [13], ring, or cut-wire [14], the approximate values of C and L can be calculated from their geometrical parameters. Figure 2. Absorption spectra of (a) sample S1 and (b) S2. Figures 2(a) and 2(b) present the simulated absorption spectra of samples S1 and S2. With the given set of parameters, the working frequency of metamaterial absorbers is about 8-12 GHz. In particular, an absorption peak of more than 97% appears at 10.5 GHz in both spectra. Their high absorption is in line with previous results [10,11], validating the magnetic origin of their absorptivity. Despite their geometrical difference, the absorption behavior is very similar to each other as expected, confirming the effectiveness of the equivalent circuit model. Nevertheless, not only the absorption intensity but also the information about their frequency scalability is important for practical applications. The geometry-frequency scalability refers to the behavior of metamaterial absorption frequency once we shrink down/up all geometrical parameters of the original design. For this purpose, the absorption behavior of selected counterparts of the proposed S1 and S2 absorbers, which operate at different frequency regime, are numerically examined according to different scaling factors relative to the sizes defined in Figs. 2(a) and 2(b). The results are presented in Fig. 3, where all the geometrical parameters are scaled down simultaneously by a factor s of 1, 0.1, 0.01, and 0.001. For the sake of simplicity, the dielectric constant of the substrate is assumed as 4+i0.008 during the simulation. It is obvious that for both S1 and S2 the ratio between the original absorption frequency (f0 = 10.5 GHz at s = 1) and scaled absorption frequency f decreases linearly with the decrease of scaling factor from 1 to 0.001. It means that geometrically scaling down a metamaterial absorber will scale up its operating frequency proportionally GHz to THz. Meanwhile, the high absorption intensity of metamaterial absorbers remains unchanged up to few tens THz (not shown here). It is even more optimistic for higher-frequency absorbers since the optical loss is more significant with used dielectric materials. Two series of differently-scaled samples correponding to S1 and S2 are fabricated to elaborate the linear geometry-frequency of metamaterial absorbers. For demonstration, the absorber samples S1 and S2 operating at 10.5 GHz are chosen as the original one with s =1. Due to the limitation of measured frequency range, other samples correspond to s=1.1 (f = 9.5 GHz) and s=0.9 (f = 11.5 GHz) are prepared and characterized as shown in Fig. 4. It can be seen that for both series of the samples the absorption frequency shifts linearly with the scaling factor s, in a good agreement with the prediction in Fig. 3. In particular, the absorption frequencies (and the bandwidth) of S1 are f=9.6 (±0.4), 10.4 Vật lý P.D. Tien, L.N. Le, , “Linear geometry-frequency scalability metamaterial absorbers.” 164 (±0.5), and 11.4 (±0.3) GHz for s = 1.1, 1.0, and 0.9, respectively. For the sample S2, the absorption frequencies (and the bandwidth) are f=9.5 (±0.4), 10.5 (±0.4), and 11.4 (±0.4) GHz for s = 1.1, 1.0, and 0.9, correspondingly. Figure 3. Linear geometry-frequency scalability of sample S1 and S2. Figure 4. Experimental and simulated absorption spectra of sample S1 and S2 according to s= 0.9, 1.0, and 1.1. Nghiên cứu khoa học công nghệ Tạp chí Nghiên cứu KH&CN quân sự, Số 49, 06 - 2017 165 The experimental geometry-frequency scalability of sample S1 and S2 for s = 0.9, 1.0, and 1.1 is plotted in Fig. 5 for comparison. The error bars indicate the corresponding fractional bandwidth of each sample. Within the examined frequency range (8-12 GHz), both S1 and S2 exhibit their linearity in geometry-frequency dependence. A small discrepancy at s = 0.9 and 1.1 could be caused by the fabrication imperfectness. 0.9 1.0 1.1 0.9 1.0 1.1 S1 S2 f 0 /f s Figure 5. Geometry - frequency dependence of sample S1 and S2. 4. CONCLUSIONS In this report, we presented a study on the geometry-frequency scalability of metamaterial absorbers. The results show that i) the absorption frequency can be precisely designed using the equivalent circuit structure, ii) the absorption frequency can be linearly scaled by geometrically shrinking up/down the metamaterial structure, and iii) the linearity of geometry-frequency scalability in metamaterial structure is experimentally examined in the range of 8-12 GHz. It is believed that the linear geometry-frequency scalability of proposed metamaterial absorbers would be useful for advanced electromagnetic probing applications. Acknowledgements: This work was supported by Academy of Military Science and Technology and by Institute of Materials Science, Vietnam Academy of Science and Technology under grant number HTTĐ 03.16. REFERENCES [1]. Electromagnetic, Mechanical, and Transport properties of Composite Materials, Rajinder Pal (CRC Press, 2014). [2]. J. B. Pendry, Contemp. Phys. 45, 191 (2004). [3]. J. B. Pendry, Phys. Rev. Lett. 85, 3966 (2000). [4]. D. Schurig, J. J. Mock, B. J. Justice, S. A. Cummer, J. B. Pendry, A. F. Starr, and D. R. Smith, Science 314, 977 (2006). [5]. N. I. Landy, S. Sajuyigbe, J. J. Mock, D. R. Smith, and W. J. Padilla, Phys. Rev. Lett. 100, 207402 (2008). [6]. X. Shen, T. J. Cui, J. Zhao, H. F. Ma, W. X. Jiang, and H. Li, Opt. Express 19, 9401 (2011). [7]. H. Tao, N. I. Landy, C. M. Bingham, X. Zhang, R. D. Averitt, and W. J. Padilla, Opt. Express 16, 7181 (2008). Vật lý P.D. Tien, L.N. Le, , “Linear geometry-frequency scalability metamaterial absorbers.” 166 [8]. Z. Fang, Y. R Zhen, L. Fan, X. Zhu, and P. Nordlander, Phys. Rev. B 85, 245401 (2012). [9]. D. T. Viet, N. V. Hieu, V. D. Lam, N. T. Tung, Appl. Phys. Express 8, 032001 (2015). [10]. N. H. Tung, L. N. Le, D. T. Viet, N. M. Thang, D. T. Anh, N. V. Duc, L. M. Thuy, N. M. Tuan, V. D. Lam, and N. T. Tung, Tạp chí Nghiên cứu Khoa học và Công nghệ Quân sự 37, 139 (2015). [11]. N. M. Thang, D. T. Anh, D. T. Viet, and N. T. Tung, Tạp chí Nghiên cứu Khoa học và Công nghệ Quân sự Số kỷ niệm 55 năm thành lập, 303 (2015) [12]. www.cst.com [13]. D. T. Viet, N. T. Hien, P. V. Tuong, N. Q Minh, P. T. Trang, L. N. Le, Y. P. Lee, and V. D. Lam, Opt. Comm. 322, 209 (2014). [14]. N. T. Tung, V. D. Lam, J. W. Park, M. H. Cho, J. Y. Rhee, W. H. Jang, and Y. P. Lee, J. Appl. Phys. 106, 053109 (2009). TÓM TẮT TƯƠNG THÍCH HÌNH HỌC - TẦN SỐ TUYẾN TÍNH CỦA VẬT LIỆU HẤP THỤ MEATMATERIALS Tương thích tuyến tính của tần số với sự thay đổi của các thông số vật lý là một ưu điểm quan trọng của vật liệu hấp thụ trong nhiều ứng dụng. Trong báo cáo này, chúng tôi nghiên cứu khả năng hấp thụ đơn giản nhưng hiệu suất cao của siêu vật liệu metamaterials, cho phép biến đổi tương cấu trúc - tần số một cách tuyến tính trong dải tần số rộng. Mẫu vật liệu hấp thụ được chế tạo bằng phương pháp quang khắc truyền thống, sau đó, đặc trưng hấp thụ được kiểm tra bằng phương pháp phân tích mạng vector trong giải tần 8-12 GHz. Kỹ thuật mô phỏng tích phân hữu hạn cũng được áp dụng để khẳng định các giá trị đo thực nghiệm. Từ khóa: Siêu vật liệu hấp thụ; Vật liệu hấp thụ; Tần số mỉcrowave. Nhận bài ngày 16 tháng 08 năm 2016 Hoàn thiện ngày 06 tháng 11 năm 2016 Chấp nhận đăng ngày 20 tháng 6 năm 2017 Địa chỉ: 1Institute of Materials Science, Vietnam Academy of Science and Technology, Vietnam; 2Hanoi University of Science and Technology, Vietnam; 3Academy of Military Science and Technology, Vietnam. *Email: thangnm@jmst.info; **Email: tungnt@ims.vast.ac.vn
File đính kèm:
linear_geometry_frequency_scalability_in_metamaterial_absorb.pdf