Gas and energy fluxes above a tropical forest in dong nai
TÓM TẮT
DÒNG NĂNG LƯỢNG VÀ KHÍ TRAO ĐỔI TRONG RỪNG NHIỆT ĐỚI
CỦA ĐỒNG NAI
Bài báo trình bày kết quả nghiên cứu, đo đạc liên tục hơn 5 năm về các dòng
năng lượng, hơi nước và khí CO2 trên hệ sinh thái rừng nhiệt đới bán thường xanh
thuộc Vườn Quốc gia Cát Tiên thông qua trạm quan trắc dòng sử dụng kỹ thuật
Eddy-Covariance đầu tiên tại Việt Nam. Những thay đổi của khí hậu trong khu vực
đã ghi nhận ở thời kỳ mùa khô 2011÷2012, 2016÷2017, thời kỳ hạn 2011÷2012,
2013÷2014 và đặc biệt là thời kỳ mùa khô 2015÷2016 khí hậu “ẩm ướt” hơn thường
lệ. Khảo sát trong số 20 trạm quan trắc dòng trong rừng nhiệt đới của khu vực, bao
gồm bức xạ thuần và tổng lượng mưa đều ghi nhận được một trong số những giá trị
cao nhất ở các thời kỳ này.
Bạn đang xem tài liệu "Gas and energy fluxes above a tropical forest in dong nai", để tải tài liệu gốc về máy hãy click vào nút Download ở trên
Tóm tắt nội dung tài liệu: Gas and energy fluxes above a tropical forest in dong nai
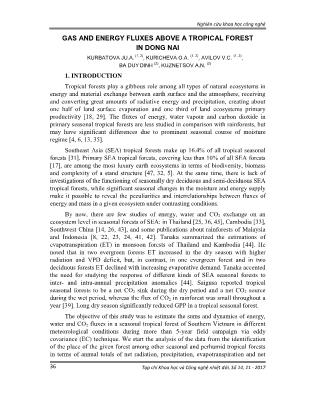
Nghiên cứu khoa học công nghệ Tạp chí Khoa học và Công nghệ nhiệt đới, Số 14, 11 - 2017 36 GAS AND ENERGY FLUXES ABOVE A TROPICAL FOREST IN DONG NAI KURBATOVA JU.A. (1, 2), KURICHEVA O.A. (1, 2), AVILOV V.C. (1, 2), BA DUY DINH (2), KUZNETSOV A.N. (2) 1. INTRODUCTION Tropical forests play a gibbous role among all types of natural ecosystems in energy and material exchange between earth surface and the atmosphere, receiving and converting great amounts of radiative energy and precipitation, creating about one half of land surface evaporation and one third of land ecosystems primary productivity [18, 29]. The fluxes of energy, water vapour and carbon dioxide in primary seasonal tropical forests are less studied in comparison with rainforests, but may have significant differences due to prominent seasonal course of moisture regime [4, 6, 13, 35]. Southeast Asia (SEA) tropical forests make up 16.4% of all tropical seasonal forests [31]. Primary SEA tropical forests, covering less than 10% of all SEA forests [17], are among the most luxury earth ecosystems in terms of biodiversity, biomass and complexity of a stand structure [47, 32, 5]. At the same time, there is lack of investigations of the functioning of seasonally dry deciduous and semi-deciduous SEA tropical forests, while significant seasonal changes in the moisture and energy supply make it possible to reveal the peculiarities and interrelationships between fluxes of energy and mass in a given ecosystem under contrasting conditions. By now, there are few studies of energy, water and CO2 exchange on an ecosystem level in seasonal forests of SEA: in Thailand [25, 36, 45], Cambodia [33], Southwest China [14, 26, 43], and some publications about rainforests of Malaysia and Indonesia [8, 22, 23, 24, 41, 42]. Tanaka summarized the estimations of evapotranspiration (ET) in monsoon forests of Thailand and Kambodia [44]. He noted that in two evergreen forests ET increased in the dry season with higher radiation and VPD deficit, but, in contrast, in one evergreen forest and in two deciduous forests ET declined with increasing evaporative demand. Tanaka accented the need for studying the response of different kinds of SEA seasonal forests to inter- and intra-annual precipitation anomalies [44]. Saigusa reported tropical seasonal forests to be a net CO2 sink during the dry period and a net CO2 source during the wet period, whereas the flux of CO2 in rainforest was small throughout a year [39]. Long dry season significantly reduced GPP in a tropical seasonal forest. The objective of this study was to estimate the sums and dynamics of energy, water and CO2 fluxes in a seasonal tropical forest of Southern Vietnam in different meteorological conditions during more than 5-year field campaign via eddy covariance (EC) technique. We start the analysis of the data from the identification of the place of the given forest among other seasonal and perhumid tropical forests in terms of annual totals of net radiation, precipitation, evapotranspiration and net Nghiên cứu khoa học công nghệ Tạp chí Khoa học và Công nghệ nhiệt đới, Số 14, 11 - 2017 37 ecosystem exchange of CO2 (NEE). Second, we describe the way of utilization of the incoming solar radiation by the forest ecosystem for generation of turbulent latent (LE) and sensible (H) heat fluxes in different meteorological conditions. Third, we draw up the characteristics of the seasonal course of gross primary production (GPP), ecosystem respiration (Reco) and resultant NEE. 2. SITE AND METHOD The meteorological and EC measurements were conducted in the newly formed Đồng Nai biosphere reserve (former Nam Cát Tiên National Park) in the Southern Vietnam. The site code in Asiaflux network list is NCT ( The nature reserve on the territory was established in 1997 [9]. The measurement site (11°27'N, 107°24'E, 150 m a.s.l.) is located in the respectively homogeneous massive of lowland mixed tropical forest. The territory has numerous streams and lakes, most of which dry up in dry season, but in wet season wide areas in the forest are flooded. The climate is Am [30], with dry period from November to April. Mean annual rainfall and temperature were 2518 mm and 26.4oC, respectively, for the period 1980÷2010 at nearby Ðong Xoài weather station [12]. In some years, usually ensuant El Niño events, precipitation is less than during the 4 month-period. According to [52], dominating at drained places are Lagerstroemia calyculata (Lythraceae), Haldina cordifolia (Rubiaceae), Tetrameles nudiflora (Datiscaceae), Afzelia xylocarpa (Caesalpiniaceae), Sterculia cf. cochinchinensis (Sterculiaceae). The forest may be characterized as primary, but probably disturbed in the second part of XX century by some human activity (selective cutting etc.). The forest has the complex structure with 4÷5 canopy sub-layers and rich biodiversity with about 80 tree species. The mean canopy height is 36÷37 m with some emergents reaching 46 m. The forest is semi-evergreen: about half of individual trees of upper sub-layers shed their foliage in a dry season. LAI is 5.1±0.9 m2 m-2 (n = 52) with ca. 0.3 m2 m-2 reduce of the values in a dry season. Soils in the site area are Dystric Skeletic Rhodic Cambisol (Clayic) according to WRB with 2÷3% of organic carbon и 0.45÷0.22% of nitrogen in the upper layer, and have a sufficient amount of phosphorus and potassium [21]. Eddy covariance [1, 2, 3, 7, 15] measurements were conducted at a 50-m height tower. NEE, LE and H were measured at 10-Hz frequency using open-path infrared gas analyser (LI-7500A, LI-COR Biosciences Inc., USA) and 3-dimentional sonic anemometer-thermometer (CSAT3, Campbell Scientific Inc., USA). 4- component radiation, precipitation, temperature and relative humidity were registered at 50 m height. Soil heat flux was measured in 4 replications. CO2 and heat storage in the air inside the forest canopy were calculated using 8-level profile measurements of CO2 concentrations and air temperature, respectively. Soil heat storage was calculated using soil temperature measurements in 3 replications. Nghiên cứu khoa học công nghệ Tạp chí Khoa học và Công nghệ nhiệt đới, Số 14, 11 - 2017 38 The database under our analysis covered the period from November 2011 to December 2016. The turbulent CO2 and heat fluxes for each 30-min period were calculated by means of EddyPro software (LI-COR Biosciences Inc., USA) with all standard EC corrections (frequency response, WPL etc.). Gaps in H, LE and NEE in 2012÷2016 made up 34%, 41% and 61%, respectively. For NEE, in night- time/day-time 79% / 31% of values were missed, respectively. Gaps in fluxes were filled using updated look-up tables method [16, 37] in Online Eddy Covariance gap- filling and flux-partitioning tool (Max-Plank Institute, Germany)1. Different gap- filling methods of Flux Analysis Tool, Japan [46] were used for fluxes uncertainty evaluation. The estimation of flux totals uncertainty depending on different gap- filling procedures for 2012 revealed the non-stability of totals and their strong dependance on night-time gaps and spikes, especially in the wettest months. As a most suitable method for gap-filling of these periods was recognized the method from [22]. The data on each step of processing (raw data, 30-minute fluxes, storages) were subjected to despiking and checking-up for physical plausibility. The ABD software by A. Deshcherevskij was used for the analysis [50, 51]. The energy balance unclosure, which is typical for EC measurements [19, 48], made up 17÷27%. Authors assumed that the turbulent fluxes had been underestimated and added the additional energy to daily, monthly and annual totals of H and LE using the Bowen ratio H/LE [15]. The prevailing winds during wet season had a predominantly south-west direction, in transitional periods a direction differed, and in dry season the winds were northern. 90% of the measured fluxes were collected from the circle with a radius of 600 m (380 m in day-time and 1360 m in night-time). For 2011÷2016, there were few 2-week gaps in the records of some parameters due to instrument malfunction, mistakes in data transfer or energy failure. Meteorological data gaps were filled using the data from other sensors or moving average diurnal variation of the adjacent data round the gap. Net radiation (Rn) in the second half of 2013 and in 2014 was recovered using the data on photosynthetically active radiation, average diurnal variation of albedo and downward long-wave radiation, air temperature in the canopy. Monthly and annual totals of precipitation in 2016 were only roughly estimated due to 4-week gap in the wet season. 3. RESULTS AND DISCUSSION 3.1. Weather conditions during the experiment Seasonality in Southern Vietnam is determined by the volatile moisture regime related to the phase of large-scale El Niño-Southern oscillation in the Pacific ocean. Wet season precipitation and temperature regime was relatively homogeneous, providing mean monthly air temperature at 50 m about 25÷27 ˚C and enough water 1 https://www.bgc-jena.mpg.de/bgi/index.php/Services/REddyProcWeb Nghiên cứu khoa học công nghệ Tạp chí Khoa học và Công nghệ nhiệt đới, Số 14, 11 - 2017 39 for vegetating. In contrast, dry seasons dramatically varied in duration and droughtiness. Dry periods of 2012÷2013 and 2013÷2014 felt within neutral phase of El Niño, but were El Niño-liked, i.e. they lasted 2.5 weeks longer than average and had less rain and cloudiness and more contrast temperature conditions (fig. 1). Fig. 1. The moisture regime in Đồng Nai: precipitation (Pr), soil water content at 5cm (SWC5cm), water vapour pressure deficit at 50 m (VPD50m) Dry period of 2015÷2016 felt with strong El Niño. Its duration was normal, but the wet season started only in mid-May, therefore April and May were as dry and hot as the few hottest months in the 30-year record of Dong Xoai station [12]. In April 30-min values of air temperature at 2 m (30.6ºС) and soil at a depth of 5 cm (28.8ºС) were the highest for the entire observation period, and absolute temperature maximum (30-min average was 40.8oC) for the whole time of observations was registered at an altitude of 30 cm. In March and April the soil water content at a depth of 5 cm was as low as 9÷11% vol., close to wilting humidity for loamy soil. By contrast, the dry season of 2016÷2017 was exceptionally wet against the backdrop of La Niña: the total precipitation for December-March 2017 was 269.2 mm (for comparison, in 2015÷2016, no rain was observed from the end of December to the middle of April). Dry period of 2011÷-2012 also coincided with La-Niña event and was wetter and milder than long-term average (rainfall from 15 of November to 15 of March was 143.4 mm). 3.2. The formation of radiation balance In contrast with pulse seasonal course of precipitation, radiation dynamic is relatively smooth with slightly higher level in the first half of a year (fig. 2). Ecosystem short-wave albedo had little seasonal changes from ca. 12.0% in the peak of dry season to ca. 10.2% in wettest months. These small changes show that the forest remain almost evergreen in terms of radiation reflectance even in the peak of dry season after a 3-month drought. The rate of net radiation to incoming radiation had the strong seasonal course, making up ca. 0.6 in February and 0.8 during wet months. 0 100 200 300 400 500 600 700 800 0 5 10 15 20 25 30 35 40 11 -2 01 1 05 -2 01 2 11 -2 01 2 05 -2 01 3 11 -2 01 3 05 -2 01 4 11 -2 01 4 05 -2 01 5 11 -2 01 5 05 -2 01 6 11 -2 01 6 Pr, mm/mon SWC, % vol. VPD, hPa Pr SWC5cm VPD50m Nghiên cứu khoa học công nghệ Tạp chí Khoa học và Công nghệ nhiệt đới, Số 14, 11 - 2017 40 Fig. 2. The downward short-wave radiation (Rs) in Đồng Nai in different years The net radiation (Rn) totals (table 1) over the forest were found to be among the highest across 21 eddy covariance stations in tropical forests world-wide, including Brazil rainforests [10, 11, 18, 20, 22, 28, 38, 40]. Only some sites in monsoon climate in Costa-Rica [27] and wet climate in Malaysia [22] and Indonesia [18, 20] in some years receive the similar or greater amount of radiation. Since the main factor that determines the amount of solar radiation in tropics is cloudiness, authors link high radiation amount in NCT with noon-time minimum of rainfall and cloudiness in Đồng Nai [12], which suggests the influence of oceanic circulation to the climate of Southern Vietnam [34]. Rn was on average 6% higher in wet half of a year in comparison with a dry half of year, creating favored conditions for vegetating. Table 1. Annual totals of fluxes at the site in Đồng Nai: net radiation (Rn), precipitation (Pr), evapotranspiration (E), net ecosystem exchange (NEE) Flux Unit Year 2012 2013 2014 2015 2016 Rn MJ m-2 y-1 4851 4694 4940 5056 4780 Pr mm y-1 2621 2634 2332 2351 2252 E mm y-1 1555 1459 1589 1556 1396 NEE gC m-2 y-1 -287 -452 -565 -350 -243 3.3. Turbulent latent and sensible heat fluxes The latent heat annual totals (table 1) were on the same level as LE values in central Amazonian rainforests despite a 4-month dry season in southern Vietnam. In a dry part of a year almost half of Rn yet spent on evapotranspiration owing to persisting transpiration of evergreen undergrowth. In 4 driest months of a year LE totals reduced only by 30% (5-year average) in comparison with 4 wettest months. Measured heat storage in soil and air accounted for 40% of morning-afternoon sensible heat flux in the hot months. 0 200 400 600 800 1 2 3 4 5 6 7 8 9 10 11 12 Rs, MJ m¯² mon¯¹ 2012 2013 2014 2015 2016 Nghiên cứu khoa học công nghệ Tạp chí Khoa học và Công nghệ nhiệt đới, Số 14, 11 - 2017 41 Fig. 3. The radation balance (Rn), latent (LE) and sensible (H) heat fluxes In contrast, in hot April 2016 turbulent heat twice exceeded the latent heat. Nevertheless, the Bowen ratio rapidly resumed to the usual wet season rate after the first rains. 3.4. CO2 fluxes Peaks of CO2 concentration in the seasonal course above the forest and inside the canopy fell at April-May and, in some years, there was a secondary peak in November-December (fig. 4). Usually, the forest was a moderate to significant sink of CO2 from the atmosphere (except for a hottest month of a year), which resulted in an annual CO2 sink in an amount of -250-550±100 gC m-2 y-1 (table 1), which is higher than mean in other tropical forests. On 4-year average, Reco and GPP in the 4 driest months made up 60% and 65% of these in 4 wettest months, respectively. The structure of the ecosystem CO2 fluxes has changed dramatically during the drough ... as contrasted with other years, when the forest was a steady net CO2 sink in all months except the hottest one. REFERENCES 1. Aubinet M., Grelle A., Ibrom A., Rannik Ü., Moncrieff J., Foken T., Kowalski A.S., Martin P.H., Berbigier P., Bernhofer C., Clement R., Elbers J., Granier A., Grünwald T., Morgenstern K., Pilegaard K., Rebmann C., Snijders W., Valentini R., Vesala T., Estimates of the annual net carbon and water exchange of forests: the EUROFLUX methodology, Advances in ecological research, 1999, 30:113-175. 2. Baldocchi D., Hicks B., Meyers T., Measuring biosphere-atmosphere exchanges of biologically related gases with micrometeorological methods, Ecology, 1988, 69:1331-1340. 3. Baldocchi D.D., Assessing the eddy covariance technique for evaluating carbon dioxide exchange rates of ecosystems: past, present and future, Global Change Biology, 2003, 9(4):479-492. 4. Billings S.A., Phillips N., Forest biogeochemistry and drought, in Forest Hydrology and Biogeochemistry, Springer, Dordrecht, 2011, p.581-597. 5. Borota J., Tropical forests: some African and Asian case studies of composition and structure, Elsevier, Amsterdam, 2012, 274 p. Nghiên cứu khoa học công nghệ Tạp chí Khoa học và Công nghệ nhiệt đới, Số 14, 11 - 2017 44 6. Bullock S. H., Mooney H. A., Medina E., Seasonally dry tropical forests, Cambridge University Press, Cambridge, 1995, 450 p. 7. Burba G., Eddy Covariance Method for Scientific, Industrial, Agricultural and Regulatory Applications: A Field Book on Measuring Ecosystem Gas Exchange and Areal Emission Rates, LI-COR Biosciences, Lincoln, 2013, 331 p. 8. Calder I.R., Wright I.R., Murdiyarso D., A study of evaporation from tropical rain forest-West Java, Journal of Hydrology, 1986, 89(1):13-31. 9. Cat Tien Biosphere Reserve (CTBR), Annual report in 2010, Đồng Nai, 2010. 10. Da Rocha H.R., Goulden M. L., Miller S. D., Menton M. C., Pinto L. D., de Freitas H. C., Seasonality of water and heat fluxes over a tropical forest in eastern Amazonia, Ecological Applications, 2004, 14(sp4):22-32. 11. Da Rocha H.R., Manzi A.O., Cabral O.M., Miller S.D., Goulden M.L., Saleska S.R., R.-Coupe N., Wofsy S.C., Borma L.S., Artaxo P., Vourlitis G., Nogueira J.S., Cardoso F.L., Nobre A.D., Kruijt B., Freitas H.C., von Randow C., Aguiar R.G., Maia J.F., Patterns of water and heat flux across a biome gradient from tropical forest to savanna in Brazil, Journal of Geophysical Research, 2009, 114(G1). 12. Deshcherevskaya O.A., Avilov V.K., Dinh Ba Duy, Tran Cong Huan, Kurbatova J.A., Modern Climate of the Cát Tiên National Park (Southern Vietnam): Climatological Data for Ecological Studies, Izvestiya, Atmospheric and Oceanic Physics, 2013, 49(8):819-838 13. Dirzo R., Young H. S., Mooney H. A., Seasonally dry tropical forests: ecology and conservation, Island Press, Washington, 2011, 408 p. 14. Dou J., Zhang Y., Yu G., Zhao S., Wang X., Song Q., A preliminary study on the heat storage fluxes of a tropical seasonal rain forest in Xishuangbanna. Science in China Series D: Earth Sciences, 2006, 49(2):163-173. 15. Eddy covariance: a practical guide to measurement and data analysis. Eds. Aubinet M., Vesala T., Papale D. Springer, Dordrecht&New York, 2012, 438 p. 16. Falge E., Baldocchi D., Olson R., Anthoni P., Aubinet M., Bernhofer C., Burba G., Ceulemans R., Clement R., Dolman H., Granier A., Gross P., Grunwald T., Hollinger D., Jensen N.-O., Katul G., Keronen P., Kowalski A., Ta Lai Chun, Law B.E., Meyers T., Moncrieff J., Moors E., Munger J.W., Pilegaard K., Rannik U., Rebmann C., Suyker A.E., Tenhunen J., Tu K., Verma S., Vesala T., Wilson K., Wofsy S., Gap filling strategies for long term energy flux data sets, Agricultural and Forest Meteorology, 2001, 107(1):71-77. 17. FAO, Global Forest Resources Assessment 2010. Main report, FAO forestry paper 163, FAO, Rome, 2010. 18. Fisher J.B., Malhi Y., Bonal D., da Rocha H., de Araujo A.C., Gamo M., Goulden M., Hirano T., Huete A.R., Kondo H., Kumagai T., Loescher H.W., Miller S., Nobre A., Nouvellon Y., Oberbauer S.F., Panuthai S., Roupsard O., Saleska S., Tanaka K., Tanaka N., Tu K.P., Von Randow C., The land-atmosphere water flux in the tropics, Global change biology, 2009, 15:2694-2714. Nghiên cứu khoa học công nghệ Tạp chí Khoa học và Công nghệ nhiệt đới, Số 14, 11 - 2017 45 19. Foken T., The energy balance closure problem: An overview, Ecological Applications, 2008, 18(6):1351-1367. 20. Huete A.R., Restrepo-Coupe N., Ratana P., Didan K., Saleska S. R., Ichii K., Panuthai S., Gamo M., Multiple site tower flux and remote sensing comparisons of tropical forest dynamics in Monsoon Asia, Agricultural and Forest Meteorology, 2008, 148(5):748-760. 21. Khokhlova O.S., Myakshina T.N., Gubin S.V., Kuznetsov A.N., Morphogenetic features of soils in the Cat Tien National Park, southern Vietnam, Eurasian Soil Science, 2017, 50(2):158-175. 22. Kosugi Y., Takanashi S., Tani M., Ohkubo S., Matsuo N., Itoh M., Noguchi S., Nik A. R., Effect of inter-annual climate variability on evapotranspiration and canopy CO2 exchange of a tropical rainforest in Peninsular Malaysia, Journal of forest research, 2012, 17(3):227-240. 23. Kumagai T., Saitoh T.M., Sato Y., Morooka T., Manfroi O.J., Kuraji K., Suzuki M., Transpiration, canopy conductance and the decoupling coefficient of a lowland mixed dipterocarp forest in Sarawak, Borneo: dry spell effects, Journal of Hydrology, 2004, 287:237-251. 24. Kumagai T., Saitoh, T.M., Sato Y., Takahashi H., Manfroi O.J., Morooka T., Kuraji K., Suzuki M., Yasunari T., Komatsu H., Annual water balance and seasonality of evapotranspiration in a Bornean tropical rainforest, Agricaltural and Forest Meteorology, 2005, 128:81-92. 25. Kume T., Takizawa H., Yoshifuji N., Tanaka K., Tanaka N., Tantasirin C., Suzuki M., Impact of soil drought due to seasonal and inter-annual variability of rainfall on sap flow and water status of evergreen trees in a tropical monsoon forest in northern Thailand, Forest Ecology and Management, 2007, 238(1):220-230. 26. Li Z., Zhang Y., Wang S., Yuan G., Yang Y., Cao M., Evapotranspiration of a tropical rain forest in Xishuangbanna, southwest China, Hydrological Processes, 2010, 24(17):2405-2416. 27. Loescher H.W., Gholz H.L., Jacobs J.M., Oberbauer S.F., Energy dynamics and modeled evapotranspiration from a wet tropical forest in Costa Rica, Journal of Hydrology, 2005, 315(1):274-294. 28. Malhi Y., Pegoraro E., Nobre A.D., Pereira M.G.P., Grace J., Culf A.D., Clement R., Energy and water dynamics of a central Amazonian rain forest, Journal of Geophysical Research, 2002, 107(D20):8061. 29. Malhi Y., The productivity, metabolism and carbon cycle of tropical forest vegetation, Journal of Ecology, 2012, 100(1):65-75. 30. McKnight T.L., Hess D., Climate zones and types: Physical geography. A landscape appreciation, Prentice Hall, Upper River, 2000, p.629. 31. Miles L., Newton A.C., DeFries R.S., Ravilious C., May I., Blyth S., Kapos V., Gordon J.E., A global overview of the conservation status of tropical dry forests, Journal of Biogeography, 2006, 33(3):491-505. Nghiên cứu khoa học công nghệ Tạp chí Khoa học và Công nghệ nhiệt đới, Số 14, 11 - 2017 46 32. Myers N., Mittermeier R.A., Mittermeier C.G., da Fonseca G.A.B., Kent J., Biodiversity hotspots for conservation priorities, Nature, 2000, 403:853-858. 33. Nobuhiro T., Shimizu A., Kabeya N., Tsuboyama Y., Kubota T., Abe T., Araki M., Tamai K., Chann S., Keth N., Year-around observation of evapotranspiration in an evergreen broadleaf forest in Cambodia. In: Forest Environments in the Mekong River Basin. Eds.: Sawada H., Araki M., Chappell N.A., LaFrankie J.V., Shimizu A., Springer, Tokyo, 2007, p.75-86. 34. Ohsawa, T., Ueda H., Hayashi T., Watanabe A., Matsumoto J., Diurnal variation of convective activity and rainfall in tropical Asia, Journal of Meteorological Society of Japan, 2003, 79:333-352. 35. Phillips O.L., Aragão L.E., Lewis S.L., Fisher J.B., Lloyd J., López-González G., Malhi Y., Monteagudo A., Peacock J., Quesada C.A., Van Der Heijden G., Drought sensitivity of the Amazon rainforest, Science, 2009, 323(5919):1344-1347. 36. Pinker R.T., Thompson O.E., Eck T.F., The albedo of a tropical evergreen forest, Quarterly Journal of the Royal Meteorological Society, 1980, 106:551-558. 37. Reichstein M., Falge E., Baldocchi D., Papale D., Aubinet M., Berbigier P., Bernhofer C., Buchmann N., Gilmanov T., Granier A., Grünwald T., Havránková, K., Ilvesniemi H., Janous D., Knohl A., Laurila T., Lohila A., Loustau D., Matteucci G., Meyers T., Miglietta F., Ourcival J.-M., Pumpanen J., Rambal S., Rotenberg E., Sanz M., Tenhunen J., Seufert G., Vaccari F., Vesala T., Yakir D., Valentini R., On the separation of net ecosystem exchange into assimilation and ecosystem respiration: review and improved algorithm. Global Change Biology, 2005, 11:1424-1439. 38. Rodrigues T.R., de Paulo S.R., Novais J.W.Z., Curado L.F.A., Nogueira J.S., de Oliveira R.G., de A. Lobo F., Vourlitis G.L., Temporal patterns of energy balance for a Brazilian tropical savanna under contrasting seasonal conditions, International Journal of Atmospheric Sciences, 2013. 39. Saigusa N., Yamamoto S., Hirata R., Ohtani Y., Ide R., Asanuma J., Gamo M., Hirano T., Kondo H., Kosugi Y., Li S.-G. Nakai Y., Takagi K., Tani M., Wang H., Temporal and spatial variations in the seasonal patterns of CO2 flux in boreal, temperate, and tropical forests in East Asia, Agricultural and forest meteorology, 2008, 148(5):761-775. 40. Shuttleworth W.J., Observations of radiation exchange above and below Amazonian forest, Journal of the Royal Meteorological Society, 1984, 110:1163-1169. 41. Takanashi S., Kosugi Y., Ohkubo S., Matsuo N., Tani M., Nik A.R., Water and heat fluxes above a lowland dipterocarp forest in Peninsular Malaysia, Hydrological Processes, 2010, 24:472-480. 42. Takanashi S., Kosugi Y., Tani M., Matsuo N., Mitani T., Nik A.R., Characteristics of the gas exchange of a tropical rain forest in Peninsular Malaysia, Phyton, 2005, 45:61-66. Nghiên cứu khoa học công nghệ Tạp chí Khoa học và Công nghệ nhiệt đới, Số 14, 11 - 2017 47 43. Tan Z., Zhang Y., Yu G., Sha L., Tang J., Deng X., Song Q., Carbon balance of a primary tropical seasonal rain forest, Journal of Geophysical Research: Atmospheres (1984-2012), 2010, 115, p.D4. 44. Tanaka N., Kume T., Natsuko Yoshifuji N., Tanaka K., Takizawa H., Shiraki K., Tantasirin C., Tangthamh N., Suzuki M., A review of evapotranspiration estimates from tropical forests in Thailand and adjacent regions. Agricultural and forest meteorology, 2008, 148:807-819. 45. Toda M., Nishida K., Ohte N., Tani M., Musiake K., Observations of energy fluxes and evapotranspiration over terrestrial complex land covers in the tropical monsoon environment, Journal of the Meteorological Society of Japan, 2002, 80(3):465-484. 46. Ueyama M., Hirata R., Mano M., Hamotani K., Harazono Y., Hirano T., Miyata A., Takagi K., TakahashiY., Influences of various calculation options on heat, water and carbon fluxes determined by open-and closed-path eddy covariance methods, Tellus B, 2012, 64(1):19048. 47. Vandekerkhove K., De Wulf R., Chin N.N., Dendrological composition and forest structure in Nam Bai Cat Tien National Park, Vietnam, Silva Gandavensis, 1993, 58:41-83. 48. Wilson K., Goldstein A., Falge E., Aubinet M., Baldocchi D., Berbigier P., Bernhofer C., Ceulemans R., Dolman H., Field C., Grelle A., Ibrom A., Law B.E., Kowalski A., Meyers T., Moncrieff J., Monson R., Oechel W., Tenhunen J., Valentini R., Verma S., Energy balance closure at FLUXNET sites, Agricultural and Forest Meteorology, 2002, 113(1):223-243. 49. WMO, 2017. World Meteorological Organization. WMO Statement on the Status of the Global Climate in 2016, WMO, Geneva, 2017, 1189. 50. Дещеревский А.В., Журавлев В.И., Никольский А.Н., Сидорин А.Я., Технологии анализа геофизических временных рядов. Часть.1. Требования к программе обработки, Сейсмические приборы, 2016a, 52(1):61-82. Часть 2. WinABD - пакет программ для сопровождения и анализа данных геофизического мониторинга, Сейсмические приборы, 2016b, 52(3):50-80. 51. Дещеревский А.В., Журавлев В.И., Никольский А.Н., Сидорин А.Я., Проблемы анализа временных рядов с пропусками и методы их решения в программе WinABD, Геофизические процессы и биосфера, 2016c, 15(3):5-34. 52. Кузнецов А.Н., Кузнецова С.П., Лесная растительность: видовой состав и структура древостоев, в сб. Структура и функции почвенного населения тропического муссонного леса (национальный парк Кат Тьен, Южный Вьетнам), под общей редакцией А.В. Тиунова, Товарищество научных изданий КМК, Москва, 2011, с. 16-43. Nghiên cứu khoa học công nghệ Tạp chí Khoa học và Công nghệ nhiệt đới, Số 14, 11 - 2017 48 TÓM TẮT DÒNG NĂNG LƯỢNG VÀ KHÍ TRAO ĐỔI TRONG RỪNG NHIỆT ĐỚI CỦA ĐỒNG NAI Bài báo trình bày kết quả nghiên cứu, đo đạc liên tục hơn 5 năm về các dòng năng lượng, hơi nước và khí CO2 trên hệ sinh thái rừng nhiệt đới bán thường xanh thuộc Vườn Quốc gia Cát Tiên thông qua trạm quan trắc dòng sử dụng kỹ thuật Eddy-Covariance đầu tiên tại Việt Nam. Những thay đổi của khí hậu trong khu vực đã ghi nhận ở thời kỳ mùa khô 2011÷2012, 2016÷2017, thời kỳ hạn 2011÷2012, 2013÷2014 và đặc biệt là thời kỳ mùa khô 2015÷2016 khí hậu “ẩm ướt” hơn thường lệ. Khảo sát trong số 20 trạm quan trắc dòng trong rừng nhiệt đới của khu vực, bao gồm bức xạ thuần và tổng lượng mưa đều ghi nhận được một trong số những giá trị cao nhất ở các thời kỳ này. Mặc dù thời kỳ mùa khô chỉ kéo dài khoảng 3,5 tháng, song các kết quả nghiên cứu đã chỉ ra tổng lượng bốc thoát hơi E và tổng sản lượng sơ cấp GPP hàng năm trong thời kỳ mùa khô tại đây gần với các dữ liệu của rừng nhiệt đới ẩm. Đối với các dòng sinh ra chủ yếu do sinh vật sống trong hệ sinh thái, sự suy giảm mùa khô có ý nghĩa quan trọng nhất đối với hô hấp hệ sinh thái hơn là đối với GPP và E, Đại lượng E ít nhạy cảm nhất với hạn hán. Rừng Nam Cát Tiên là một bể chứa CO2 quan trọng từ khí quyển giai đoạn 2012÷2015, ngoại trừ những tháng nóng nhất của năm. Điều kiện nóng và khô bất thường trong tháng 4 và tháng 5 năm 2016 đã gây ra sự gia tăng dòng năng lượng hiển nhiệt và hô hấp của hệ sinh thái. Sau cơn mưa đầu tiên, trong khi các dòng năng lượng nhanh chóng quay trở lại trạng thái thông thường của thời kỳ mùa mưa thì rừng vẫn đóng vai trò là nguồn phát CO2 vào khí quyển trong khoảng thời gian thêm 1,5 tháng. Keywords: Eddy covariance, seasonally dry tropical forest, Đồng Nai biosphere reserve, net ecosystem excange, evapotranspiration. Nhận bài ngày 24 tháng 9 năm 2017 Hoàn thiện ngày 02 tháng 11 năm 2017 (1) A.N.Severtsov Institute of Ecology and Evolution, RAS (2) Vietnam - Russian Tropical Center
File đính kèm:
gas_and_energy_fluxes_above_a_tropical_forest_in_dong_nai.pdf